RESEARCH TOPICS
ON/OFF regulation of helix inversion:
-- Control of helix inversion rate by molecular recognition
Helical molecules that can undergo reversible helicity inversion between the left- and right-handed forms are referred to as a "dynamic helical molecule". If the left- and right-handed forms are enantiomers of each other, these two forms exist in a 1:1 ratio as an equilibrated racemic mixture (Figure 1 left). However, one of the two forms dominates in case these structures are interacting with a chiral molecule (Figure 1 right). This equilibrium shift is known as dynamic helicity control. Based on this concept, we can easily obtain a biased helix from a racemic mixture.
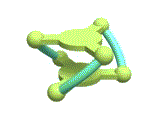
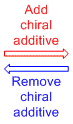
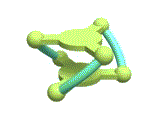
[Hover to see the helix inversions for both cases.]
Then, can we keep the biased helicity induced by a chiral source after removal of the chiral source? The answer is "NO". The biased helicity, "chiral information" of the helix, will be lost after removal of the chiral source, because the helix is a "dynamic helix". If we want to keep the helicity as biased, we need to introduce some mechanisms that can retard helicity inversion after the equilibrium shift. Thus, we focused on a combination of a dynamic helix inversion and a host-guest complexation (Figure 2). We designed a trinuclear nickel(II) complex, which has a cage-like structure with three square planar [Ni(saloph)] moieties connected with two pivotal benzene rings (Figure 3).
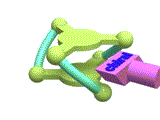
[Hover to animate]
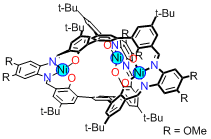
The X-ray crystallographic analysis (Figure 4 left) revealed that the complex adopted a triple-helical structure resulted from the rigid 1,3,5-triphenylbenzene scaffolds. The binding studies of showed that this trinickel(II) metallocryptand can recognize various kinds of cationic guests. In particular, the metallocryptand can strongly encapsulate a guanidinium ion (H2N)2C=NH2+ in the cavity (Figure 4 right). The metallocrpytand recognized chiral ammonium ions, resulting in a biased helicity which was observable as induced CD signals.
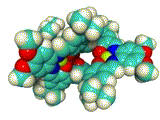
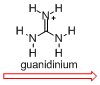
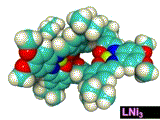
[Hover to animate]
As described above, the trinuclear metallocryptand can recognize a guanidinium ion very strongly
and the helicity of the metallocryptand was biased upon the recognition of a chiral ammonium ion.
Based on these facts, we investigated to see if the biased helicity by a chiral ammonium ion could be kept by encapsulation of guanidinium ion.
The CD spectroscopic investigation clearly showed that the biased helicity remained unchanged
while the chiral ammonium guest was immediately replaced with a guanidinium ion (half life within 12 sec).
The CD signal gradually decayed with a half life of 2200 sec.
This means that the helicity inversion was retarded by a factor of more than 180 after encapsulation of a guanidinium ion.
Consequently, this metallocryptand worked as a helicity storage system based on the guest exchange (Figure 5).
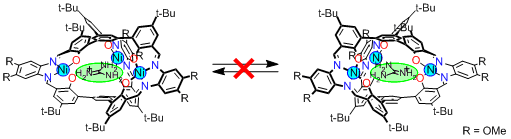
When we develop a functional molecular system based on dynamic helicity inversion,
both helicity control efficiency (ratio of the left- and right-handed forms) and response speed are the important factors.
As for the helicity control efficiency, higher is better.
It is preferable that an external stimulus can completely shift the helix inversion equilibrium to yield a one-handed helix.
However, it is not so simple to discuss the response speed, that is, the desirable helicity inversion rate.
For example, faster helix inversion is desirable when we need to record chiral information.
On the other hand, slower helix inversion is desirable when we use the chiral functions,
because the helix inversion might cause loss of the chiral information.
From this viewpoint, the helicity inversion system like the nickel(II) metallocryptand,
in which we can control the helix inversion rate in an ON/OFF fashion,
is useful for the development of switchable chiral functional systems.
[Reference]
“Perfect encapsulation of guanidinium ion in a helical trinickel(II) metallocryptand
for efficient regulation of helix inversion rate”
Akine, S.; Miyashita, M.; Piao, S.; Nabeshima, T.
Inorg. Chem. Front. 2014, 1, 53-57
(invited article in the first issue of this journal).
doi:10.1039/C3QI00067B